-
Chem 220 -Organic Chemistry
Problem Set 6
Solution Set
Chapter 7, Structure and Synthesis of
Alkenes
Due: Monday, October 18, 2010
Notice:
For those of you who are using the 6th edition of the
textbook, you can learn about olefin metathesis by
clicking
here.
1. Read Degree
(Elements) of Unsaturation. How
many degrees of unsaturation are present in
C16H13ClN2O? Draw two
structures, one cyclic, the other acyclic, that have the
number of degrees of unsaturation you determined and that is
necessarily in agreement with the formula.
We begin with
C16H13ClN2O.
Dropping O gives
C16H13ClN2.
Drop Cl, add one H:
C16H14N2
Each N is worth CH;
therefore, C18H16
The most saturated
C18 hydrocarbon is C18H38.
(38-16)/2 = 11 degrees of unsaturation.
If you like formulas,
let's derive one from what was done above.
The number of
hydrogens in the most saturated hydrocarbon must be 2C + 2
less the number hydrogens, halogens and nitrogen equivalents
all divided by 2. Since the number of carbons in the most
saturated hydrocarbon is increased when nitrogen is replaced
by CH, one has D.U. = {[2(C+N)+2]-(H+X+N)}/2. This
equation can be reduced to D.U. = C-((H+X-N)/2)+1. For the
case at hand, D.U. = 16-(12/2)+1 = 11.
Two structures are
below. The cyclic structure happens to be valium (About its
inventor, Leo
Sternbach).
|
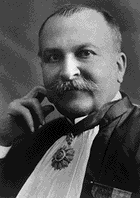
Paul
Sabatier
1912 Co-Nobel Prize in Chemistry
Hydrogenation by Metal Catalysis
|
2. a) Determine the heat of formation of
3-methyl-1-pentene by using the heat
of formation tables to determine
typical heats of hydrogenation for monosubstituted alkenes.
Show work. The
hydrogenation product of 3-methyl-1-pentene is
3-methylpentane, ΔHfo = -41.0
kcal/mol. Looking at the ΔHfo of
typical 1-alkenes, one can see: 1-butene (ΔHfo
= 0 kcal/mol), 1-pentene (ΔHfo
= -5.0 kcal/mol) and 1-hexene (ΔHfo
= 10.2 kcal/mol). Notice each one differs by ~5 kcal/mol.
Their respective alkanes are: n-butane (ΔHfo
= -30.0 kcal/mol), n-pentane (ΔHfo
= -35.1 kcal/mol) and 1-hexene (ΔHfo
= -39.9 kcal/mol). The heats of hydrogenation of each of
these is,respectively, the difference in the heats of
formation: -30.0 kcal/mol, -30.1 -30.0 kcal/mol and -30.0
kcal/mol-29.7 -30.0 kcal/mol. The average is -29.9 kcal/mol.
Therefore, the ΔHfo of
3-methyl-1-pentene is x = -41.0 -(-29.9) = -11.1 kcal/mol.
The reported ΔHfo of
2-methyl-1-butene is -6.1 kcal/mol. Add a methylene to this
structure (-5.0 kcal/mol) and you obtain ΔHfo
= -11.1 kcal/mol for 3-methyl-1-pentene.
b) Calculate the heat of
hydrogenation of (E)-and
(Z)-3-methyl-2-pentene. Show work.
From the Heats of
Formation Table, (E)-3-methyl-2-pentene is ΔHfo
= -15.2 kcal/mol and (Z)-3-methyl-2-pentene is ΔHfo
= -14.8 kcal/mol. The heat of formation of 3-methylpentane
is ΔHfo = -41.0 kcal/mol. The
heat of formation of the (E)-isomer is -41.0 - (-15.2) =
-25.8 kcal/mol. The heat of formation of the (Z)-isomer is
-41.0 - (-14.8) = -26.2
kcal/mol.
c) Use a diagram to illustrate that the difference in the
heat of hydrogenation of the two geometrical isomers in 2b
is equal to the difference in their heats of formation.
Which isomer is more stable based upon the heats of
formation? Why?
From the
diagram on the right, ΔHfo(E)
+ HHo(E) = ΔHfo(Z)
+ HHo(Z). Therefore, ΔHfo(E)
- Hfo(Z) = ΔHHo(Z)
- HHo(E). The (E)-isomer is
more stable. more negative heat of formation; lower
heat of hydrogenation.
d) There is only one
monosubstituted alkene having the carbon skeleton
of the (E)-and (Z)- isomers in 2b.
What is its structure? Assuming that ΔGo
= ΔHo, which of the three isomeric
alkenes would dominate in an equilibrium mixture?
How much heat is liberated in the isomerization of
the monosubstituted alkene to the
(E)-isomer? Show work. Add the
monosubstituted alkene to your diagram in 2c and
illustrate the heat of isomerization.
The
isomer is 3-methyl-1-pentene, the answer to part
a). The (E)-isomer would dominate in an equilibrium
mixture because it is the most stable. The heat of
isomerization equals -15.2 - (-11.1) = -4.1
kcal/mol.
|
|
|
3. a) Using the standard values for the relative reactivity
of 1o, 2o and 3o C-H bonds
toward free radical chlorination, determine the relative
amount of the four possible monochlorides formed from the
chlorination of [2.2.1]-bicycloheptane.
Positions 1, 2, 4 and
5 are all the same type of CH2 [8 x 4.5 =
36]. They lead to the same radical. Position 7 is a
unique CH2 [2 x 4.5 = 9]. Positions 3 and
6 are bridgehead hydrogens [2 x 5.5 = 11]. Dividing
each of these products by the sum (56) gives the relative
amounts: 16%, 64% and 20%, respectively.
b) The experimental result is: 1,
72%; 2, 25%; 4, 3%; 3, 0%. Why is no
bridgehead isomer formed even though tertiary C-H bonds are
highly reactive?
The radical from
the the bridgehead hydrogen cannot become planar.
c) There are two types of
secondary radicals formed. What is their relative
reactivity?
[Hints: Measure the
C2-C1-C6 and the
C3-C7-C6 bond angles in
norborane on the right in which the radical is formed on the
central carbon. How do these values compare with
cyclopentane?
The chlorination of cyclobutane is slower than the
chlorination of cyclopentane.]
97/3 = 32.3, which
is the sum of exo + endo divided by 3% for
4. This selectivity is eight times
greater than 36/6 = 4 calculated in part a). Since the C-C-C
bond angle in cyclopentane is 104o and in
cyclobutane it is 88o and cyclobutane is slower
to chlorinate, perhaps this is part of the problem.
Measuring the angles in norbornane, the
C2-C1-C6 is
103o and the
C3-C7-C6 bond angle
is 93.5o.
d) At what point in the radical chain are the endo and exo
isomers formed? Why does the exo isomer dominate? Explain
and illustrate.
The radical formed
at C1, C2, C4 or
C5 in the first propagation step, reacts with
chlorine in the second propagation step proximate to
C7, leading to the exo isomer, or distal to
C7, leading to the endo isomer. Imagine a plane
through carbons 6-1-2-3. A reaction is less hindered
proximate to the the smaller methano- bridge (C7)
than to the ethano- bridge (C4-5). This is why
the exo isomer dominates.
e) Are the four monochlorides optically
active, racemic, or achiral?
Exo and endo are
racemic; the other two are achiral.
|
How
to manipulate Jmol structures.
Larger
Version
|
4. The major product B from free
radical monobromination of alkane A
(C6H14) readily reacts with water to
form C, C6H14O. Treatment of
bromide B with KOH in ethanol produces two, and only
two, compounds, D and E. Compound D
liberates 1.6 kcal/mol more heat upon hydrogenation to
A than does E. What are the structures
A-E? What is the heat of isomerization of D to
E? Illustrate and explain. [Hint: What are
the structures possible for A?]
There are
five possible structures, 1-5,
for the acyclic, saturated alkane
A. Since bromide
B reacts readily with water
(SN1) to form alcohol
C, structures
1 and 5
are eliminated. Structure 3 is
also eliminated because E2 elimination of
3-bromo-3-methylpentane could give three alkenes:
2-ethyl-1-butene and (E)- and
(Z)-3-methyl-2-pentene. That leaves
6 and 7
from 2 and
8 and 9
from 4 for the structures of
D and E.
Lookup the heats of formation of
4, 8 and
9 in the the
Heats
of Formation
Tables.
From these data, one calculates the difference in
the heat of hydrogenation as 1.1 kcal/mol, which
isn't the value 1.6 kcal/mol. Alternatively, Table
7-1 in Wade gives the difference as 1.4 kcal/mol.
(there is some discrepancy here.) Neither
6 nor 7
appear in the heats of formation table but we can
look at lower homologs and calculate the heats of
formation using the 5.0 kcal/mol/CH2.
Thus, 2-methyl-1-butene (ΔHfo
= -8.4 kcal/mol) gives ΔHfo
= -13.4 kcal/mol for 7 while
2-methyl-2-butene (ΔHfo
= -10.0 kcal/mol) affords ΔHfo
= -15.0 kcal/mol for 6. The
difference is 1.6 kcal/mol. [There is actually
no need to include the 5 kcal/mol correction
because the difference would be the same.]
Using Table 7-1 in Wade, a value of 1.6 kcal/mol is
obtained. So, A =
2-methylpentane; B =
2-bromo-2-methylpentane; C =
2-methyl-2-pentanol; D =
2-methyl-1-pentene (7);
E = 2-methyl-2-pentene
(6). D,
with a less negative heat of formation than
E, liberates more heat on
hydrogenation.
|
|
|
5. In 1968 Wiberg
and Fenoglio determined the heat of combustion of
cyclopropene: -485.0 kcal/mol. Determine the heat of
hydrogenation of cyclopropene to cyclopropane. Explain and
illustrate with a diagram. What is needed is the heat of
formation of both cyclopropene and cyclopropane.
The alkene's heat
of formation is calculated as +66.2 kcal/mol in the usual
way. See left side of diagram on the right. The heat of
formation of cyclopropane comes from the
Heats
of Formation
Tables.
The difference in heats of formation is the heat of
hydrogenation: -53.5 kcal/mol. In the chart below the heats
of formation of simple cycloalkenes and cycloalkanes are
listed. The heats of hydrogenation are computed.
Cyclopropene's double bond is really distorted (bond
angles). Note that the heat of hydrogenation of cyclohexene
is no different from the heat of hydrogenation of
(Z)-2-butene.
|
|
6. Two stereoisomers, A and
B, absorb one equivalent of hydrogen upon catalytic
hydrogenation to form cyclooctane. Compound A, which
is capable of resolution, liberates 34.5 kcal/mol of heat
while B liberates 24.3 kcal/mol of heat.
a) What are the structures of A and B ?
Since
A and B both
absorb only one equivalent of hydrogen to form cyclooctane,
they must be cis- and trans-cyclooctene. trans-Cyclooctene
is more strained than cis-cyclooctene because a chain of six
methylene groups is the minimum length to span a double bond
that is trans. It gives off more heat on hydrogenation.
Therefore, A is trans;
B is
cis.
b) What are the heats of formation of A and B
? The heat of
formation of cyclooctane is from Heats
of Formation
Tables.
The heats of hydrogenation are given. The differences gives
the respective heats of formation.
c) What is the difference in
strain energy between A and B
? The
difference between the heats of formation: 10.2
kcal/mol.
d) What is the difference in the
heat of combustion between A and B?
From the diagram
on the right it is obvious than the difference in the heats
of combustion is the same as the difference in the strain
energy of the two.
e) Why is A capable of
resolution? The non-superimposable mirror images of
trans-cyclooctene do not interconvert.
It is difficult
for the the vinyl hydrogens to pass through the ring to
racemize. Link
to Jmol structures here.
|
|